Courtney M. Dumont, Ph.D.
Assistant Professor, Department of Biomedical Engineering
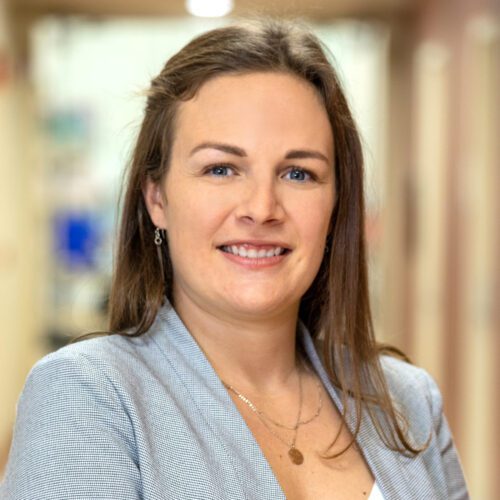
The Miami Project to Cure Paralysis
1095 NW 14th Terrace (R-48)
Miami, FL 33136
(305) 243-6142
Biography
Research Interests
Areas Of Research
Publications
More
Courtney Dumont is an Assistant Professor in the Department of Biomedical Engineering. Dr. Dumont leads the Neural Stem and Tissue Engineered Microenvironments (NeuSTEM) group, working at the interface of neural, vascular, and immune engineering to overcome barriers that arise after injury to the nervous system. Dr. Dumont received her PhD in biomedical engineering from Rensselaer Polytechnic Institute in 2014, investigating the role of altered fluid mechanics on vascular cell regulation of the neural stem cell niche.
She completed her postdoctoral work at the University of Michigan, where she developed a modular biomaterial system for gene, drug, and stem cell delivery to promote repair after spinal cord injury. In 2020, Dumont was awarded the inaugural Junior Frost Fellow Award in chemistry for her targeted drug delivery strategies following nerve injury. Her lab continues to develop new biomaterial, stem cell, and drug delivery strategies that address intrinsic and extrinsic barriers to spinal cord repair and works closely with members of The Miami Project to Cure Paralysis to continue to redefine our understanding of the pathophysiology after injury and identify new therapeutic targets.
Biomaterial Approaches for Spinal Cord Repair
Injuries to the nervous system resulting in a gap defect can have devasting functional consequences, particularly within the spinal cord. Biomaterial intervention is often seen as a promising therapy as these materials can fill the void left by the damaged tissue, bridge opposing ends of the injury site, and support cellular infiltration. Our team investigates and develops the combined benefits of bridging technologies that guide regenerating axons, microporous structures to facilitate support cell infiltration and integration of the scaffold into the surrounding tissue, mechanical properties that can more closely mimic that of the soft spinal cord tissue, and utility of biomaterials as a cellular, drug, and/or gene therapy depot. Using a modular hydrogel system we can promote axon guidance through tubes that can be cut to size and packed as needed into the injury site. Our design serves as a hybrid strategy that incorporates the best features of hydrogels, namely their ability to conform to an injury site, and pre-formed or molded scaffolds that provide topographical guidance. We are currently exploring the integration of nanoscale biomaterials with our scaffolds to improve on-demand pharmacological agent localization within the injury site as well as cytokine scavenging capabilities to reduce inflammation. Together, these strategies will help to move the field of neural tissue engineering forward, while also providing essential information about the underlying cellular biology and injury pathophysiology.
On-Demand Local Drug Delivery for Tissue Engineering
Nanoscale therapeutics, including nanoparticles, provide protection for sensitive pharmacological agents administered within the body. Moreover, nanoparticles can be designed to include targeting moieties to increase accumulation in target tissues. While the sophistication of nanoparticle technologies has advanced substantially, their targeting moieties still rely heavily on proteins expressed ubiquitously throughout a tissue (e.g. not injury specific). Additional challenges exist with delivery to the central nervous system due to limited vascular accessibility caused by the blood-brain barrier. Our team is working to address these challenges through the development of a biomaterial-targeting nanoparticle system that can serve as an on-demand local drug delivery platform to promote tissue repair. Our system has been designed using a targeting delivery vehicle and a receptor-beacon comprised of highly tunable, synthetic materials that can be broadly applied to deliver therapeutics to any tissue. Feasibility of this system has been demonstrated in a cervical spinal cord injury model for up to 4 weeks after injury, with early pilot data demonstrating nanoparticle accumulation at 6 months post-injury. The successful completion of this project will result in a drug delivery platform that can facilitate on-demand, local drug delivery for several months after injury and facilitates the localization of repeated dosages at the selected injury site.
Neural Stem Cell-Mediate Repair
Neural stem cells (NSCs) are a promising therapeutic option to promote regeneration of damaged spinal tissue. However, the regenerative potential of NSCs is severely limited by their poor survival after transplantation into the injury. Because of their poor survival, the long-term benefits of increased NSC transplant survival have not been able to be evaluated. Our team investigates biomaterial-based strategies to bolster transplant survival either alone or in combination immunomodulatory therapies. In addition to improving transplant survival, our team is interested in studying NSC-mediated mechanisms of repair, namely the cross-talk that occurs between NSCs and immune cells within the injury site. Using encapsulating biomaterials, we can isolate NSCs within the injury to create a physical and biochemical barrier between NSCs and immune cells. Through careful tuning of the biomaterial, we can tune the diffusion of molecules of interest to better understand NSC-immune communication at the injury site with the goal of developing more effective NSC therapies for tissue repair.
PROFESSIONAL AFFILIATIONS/MEMBERSHIPS
- Biomedical Engineering Society
- Society for Biomaterials
- International Society for Stem Cell Research